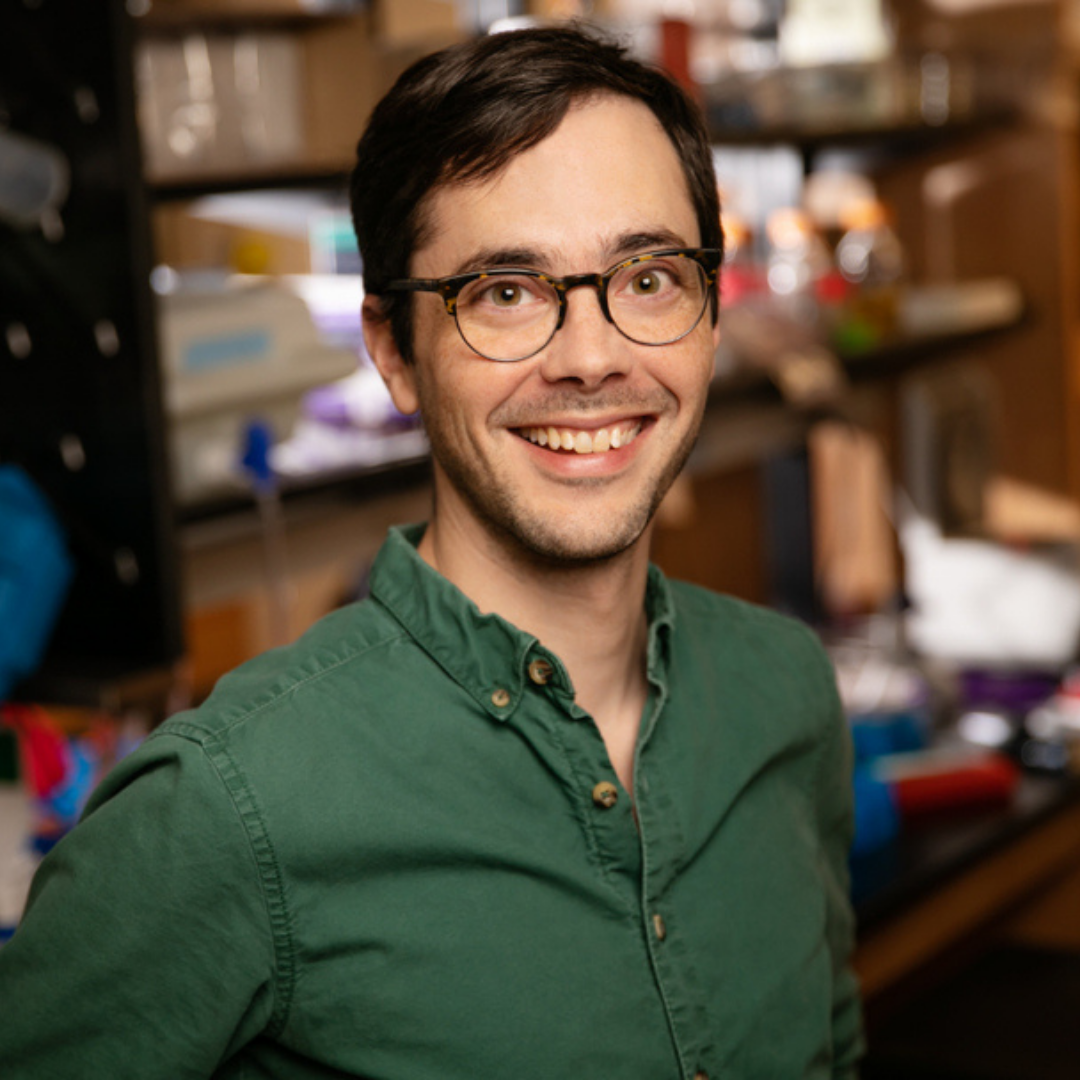
When Assistant Professor of Molecular and Cellular Biology Christopher Brooke was three years old, his mom bought him a book about marine creatures.
“For whatever reason—I think just because I thought the animals were really weird—I was super into it,” Brooke recalled. This childhood fascination with biology turned viral at college, where he worked in a lab researching influenza and fell in love with the environment.
“People coming in and working to satisfy their own curiosity—that just seemed like a really nice way to make a living ... So I was hooked at that point.”
After completing a Ph.D. focusing on how the immune system fights off viruses, Brooke turned back to flu, “because it’s kind of shocking how little we know about this virus that kills so many people and costs so much money, even though we’ve been studying it for decades.”
Now, Brooke leads his own lab at the School of Molecular and Cellular Biology. One of its goals: understanding how influenza adapts, transmits and replicates.
At its most basic, influenza is “a sort of code for propagating itself,” Brooke said. The virus is made up of eight ribonucleic acid (RNA) molecules. When it infects a cell, it rewires that cell to turn it into a factory producing more RNA molecules as well as the machinery needed to turn those molecules into new flu particles. Those particles then move on to another cell and the process starts over.
What makes the virus so dangerous is its remarkable adaptability. Worldwide, there are between 300,000 and 650,000 flu fatalities each year; in the United States alone, upwards of $10 billion is spent annually on tackling influenza and its effects.
Adaptability refers to the ability of the virus to evolve to thrive in new environments or overcome selective pressures—for example, “asking [the virus] to replicate in a new species or putting it in a human that has some degree of immunity,” Brooke explained.
“That selective pressure will select for variants within the starting virus population that have different genomic sequences that make them better able to handle whatever that selective pressure is.”
In other words, when the virus infects people with a high level of immunity, there is selection for traits that help it escape that immunity. Thus, a new strain is born.
And non-human strains pose a graver threat.
“We typically have some degree of pre- existing immunity against human influenza strains, [but] animal (or zoonotic) strains—we have no immune protection against them,” said Brooke.This means that if a new strain jumps species, it can replicate more efficiently and lead to a pandemic.
The Brooke Lab focuses on influenza A, the strain that affects humans and some other mammals, including birds and swine.
By expanding our understanding of the mechanisms behind the virus’ ability to adapt and transmit, Brooke hopes that his team might contribute to the creation of a universal flu shot.
“If we could develop a vaccine that actually worked in the same way that the polio vaccine or the measles vaccine works—especially if it’s effective and can actually eradicate the virus— that would be a pretty significant benefit for mankind.”
Findings in a forthcoming paper may provide one clue to fighting influenza on a broader scale.
The textbook image of a flu virus, Brooke explained, shows eight identical genome segments. But when he and his colleagues analyzed populations of flu virus particles, they found that they were, in fact, far from genetically identical.
“You can really think of a population of influenza viruses as a collection of incomplete particles that carry fractions of the genome. They have to work collectively to replicate,” Brooke said.
That is to say, once the virus has entered a cell, only a minority of the viral particles—from as low as one to no more than 30 percent—can replicate on their own. These semi-infectious particles (a term coined at the lab) can infect a cell but they cannot multiply without the help of other particles.
What might this mean for a potential vaccine?
“If the virus really does have to work together as a collective to replicate efficiently, [then maybe] there are certain constraints that could potentially be targeted.”
Finding those constraints means detecting patterns within multiple flu strains.
“Where our vaccines and our immune system currently target the virus, it is very tolerant of mutations, so it’s able to change and escape detection,” said Brooke. But if researchers can identify parts of the virus that are not as adaptable, then it might be possible to develop treatments that attack those areas.
According to Brooke, “figuring out where to target the virus ... and then figuring out how to instruct the immune system to target those regions are really the two breakthroughs that need to be made. I think that’s the goal of the field and it might take 10 or 20 years or more, because these are really difficult problems.”
Collaboration in the name of curiosity is still something Brooke enjoys.
Working with undergraduates, post-docs and graduate students in his lab is “very enjoyable, because they bring different perspectives, they have different ideas that you would never have,” he said. “It’s really satisfying to be able to watch [them] develop as scientists right in front of you.”
And even if influenza were eradicated tomorrow, Brooke’s curiosity would not be extinguished. There are, after all, plenty of other viruses that he finds just as fascinating.
“They’re super weird and I’m still constantly a little bit freaked out by them,” Brooke said. “I find them to be continuously surprising and strange, and that keeps me interested.”