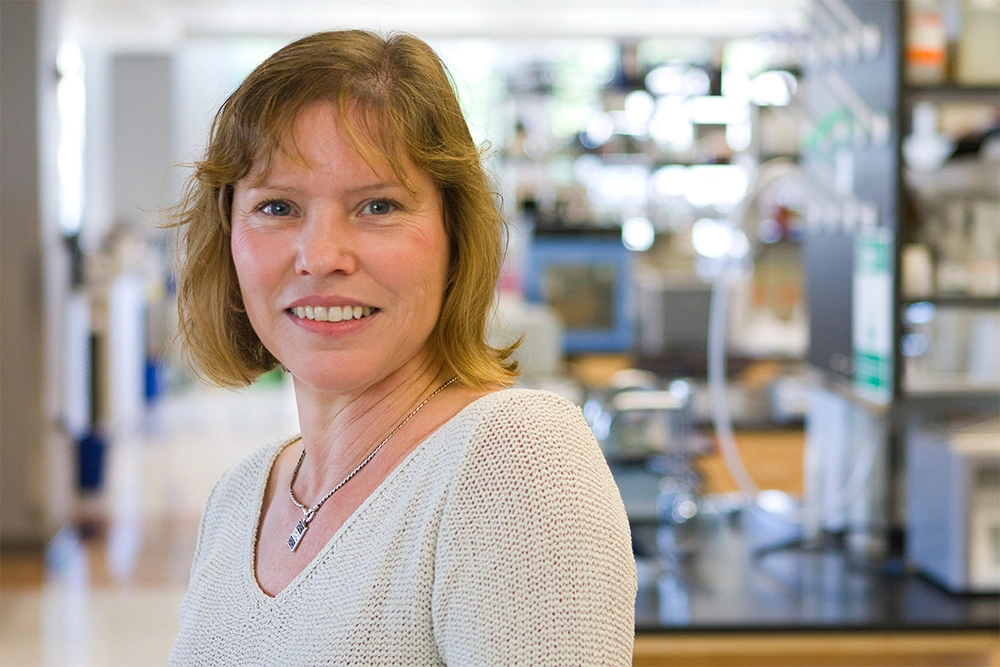
Mice have a reputation for timidity. Yet when confronted with an unfamiliar peer, a mouse may respond by rearing, chasing, grappling, and biting—and come away with altered sensitivity toward future potential threats.
What changes in the brain of an animal when its behavior is altered by experience? Research at the University of Illinois led by Professor of Cell and Developmental Biology Lisa Stubbs is working toward an answer to this question by focusing on the collective actions of genes. In a recent Genome Research publication, Stubbs and her colleagues identified and documented the activity of networks of genes involved in the response to social stress.
“The goal of this study was to understand the downstream events in mice, and how they are conveyed across interacting brain regions . . . how they might set the stage for emotional learning in response to social threat,” said Stubbs. Answers to these questions could help scientists understand how the brains of other animals, including humans, generate social behavior, as well as what goes wrong in disorders of social behavior.
The new results are part of a large-scale research project funded by the Simons Foundation that is headed by Stubbs and includes many of her coauthors, including first authors Michael Saul and Christopher Seward.
An aggressive encounter between two mice is just one strand of the web of interactions that connects a population of social animals. Like individuals in a community, the genes in a genome cannot be completely understood until their relationships to one another are examined in context, including how those relationships may change across different tissues and over time.
Stubbs’ team wanted to gather information that would allow them to construct this type of comprehensive gene network to reflect how the brain of a social animal responds to an aggressive encounter. They staged a controlled encounter between pairs of mice; one mouse in its home cage, and a second, unfamiliar mouse introduced behind a screen. The presence of the intruder mouse created a social challenge for the resident mouse, while the screen prevented a physical encounter.
The researchers then quantified the activity of genes in several different regions of the brain associated with social behaviors—the frontal cortex, hypothalamus, and amygdala—and at several time points in the two hours following the encounter. In analyses of the resulting data, they looked for groups of genes acting together. In particular, they sought to identify transcription factors, genes whose protein products help control other genes, that might be orchestrating the brain’s molecular response.
Stubbs was excited to discover that the results mirrored and expanded upon previous work in other species by collaborators, including work by the laboratory of Director Gene Robinson in honey bees.
“As we examined the regulatory networks active in the mouse brain over time, we could see that some of the same pathways already explicated in honey bees . . . were also dysregulated similarly by social challenge in mice,” she said. “That cross-species concordance is extremely exciting, and opens new doors to experimentation that is not being pursued actively by other research groups.”
Among the genes responding to social challenge were many related to metabolism and neurochemical signaling. In general terms, it appeared that cells in the brains of challenged mice may alter the way they consume energy and communicate with one another, changes that could adjust the neural response to future social experiences.
The researchers looked for associations between genes’ responses to social experience and their epigenetic state. How different regions of DNA are packaged into the cell (sometimes referred to as chromatin structure) can influence the activity of genes, and so-called epigenetic modifications, changes to this structure, help to modify that activity in different situations.
“We found that the chromatin landscape is profoundly remodeled over a very short time in the brain regions responding to social challenge,” said Stubbs. “This is surprising because chromatin profiles are thought to be relatively stable in adult tissues over time.” Because such changes are stable, they are sometimes hypothesized to reinforce long-term behavioral responses to experience.
Stubbs and her colleagues hope that by identifying genomic mechanisms of social behavior that are basic enough to be shared even between distantly related animal species, they can discover which biological mechanisms are most central.
“The most exciting thing in my view is using [comparisons between species] to drill through the complex response in a particular species to the ‘core’ conserved functions,” she said, “thereby providing mechanistic hypotheses that we can follow by exploiting the power of genetic models like the mouse.”