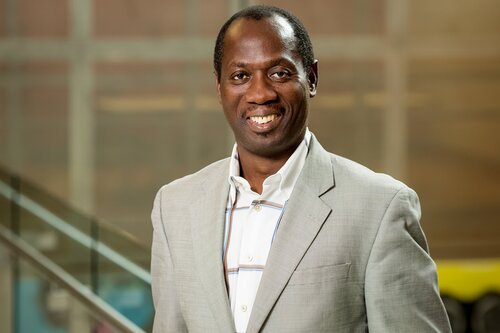
Contact Information
1206 West Gregory Drive
MC-195
Urbana, IL 61801
Research Interests
Research Topics
Archaea, Biofuels, Enzymology, Genomics, Host-Pathogen Interactions, Molecular Evolution, Protein Structure, Protein-Nucleic Acid Interactions
Research Description
Molecular biology and biochemistry of archaeal DNA replication; host-microbiome interactions; enzymes of plant cell wall hydrolysis
The research of the Cann lab focuses on three areas:
- DNA replication in the archaeal domain of life
- Host-microbiome interactions and their effects on health and disease
- Enzymes of importance to biofuel production
Isaac Cann is a member of two themes at the Carl R. Woese Institute for Genomic Biology (IGB), and his research is carried out at the space shared by his affiliated themes [Microbiome Metabolic Engineering (MME) and Biocomplexity (BCXT)] with others at the IGB.
Education
B.Sc (Hons) Animal Sciences, University of Ghana 1986
M.S. (Rumen Microbiology), Mie University, Japan, 1991
Ph.D. (Rumen Microbiology), Mie University, Japan, 1994
Postdoctoral (Anaerobic Microbiology), Department of Animal Sciences, University of Illinois, 1994-1997
Awards and Honors
National Science Foundation CAREER Award 2003
Fellow, Center for Advanced Study 2005
Excellence in Guiding Undergraduate Research Award (UIUC) 2012
Paul A. Funk Award, College of ACES 2014
Additional Campus Affiliations
Professor, Animal Sciences
Professor, Nutritional Sciences
Professor, Carl R. Woese Institute for Genomic Biology
Affiliate, Microbiology
Professor, Center for East Asian and Pacific Studies
Recent Publications
Tsuruga, T., Fujimoto, H., Yasuma, T., D'Alessandro-Gabazza, C. N., Toda, M., Ito, T., Tomaru, A., Saiki, H., Okano, T., Alhawsawi, M. A. B., Takeshita, A., Nishihama, K., Takei, R., Kondoh, Y., Cann, I., Gabazza, E. C., & Kobayashi, T. (Accepted/In press). Role of microbiota-derived corisin in coagulation activation during SARS-CoV-2 infection. Journal of Thrombosis and Haemostasis. https://doi.org/10.1016/j.jtha.2024.02.014
Fridman D'Alessandro, V., D'Alessandro-Gabazza, C. N., Yasuma, T., Toda, M., Takeshita, A., Tomaru, A., Tharavecharak, S., Lasisi, I. O., Hess, R. Y., Nishihama, K., Fujimoto, H., Kobayashi, T., Cann, I., & Gabazza, E. C. (2023). Inhibition of a Microbiota-Derived Peptide Ameliorates Established Acute Lung Injury. American Journal of Pathology, 193(6), 740-754. https://doi.org/10.1016/j.ajpath.2023.03.003
Nishiwaki, R., Imoto, I., Oka, S., Yasuma, T., Fujimoto, H., D’Alessandro-Gabazza, C. N., Toda, M., Kobayashi, T., Osamu, H., Fujibe, K., Nishikawa, K., Hamaguchi, T., Sugimasa, N., Noji, M., Ito, Y., Takeuchi, K., Cann, I., Inoue, Y., Kato, T., & Gabazza, E. C. (2023). Elevated plasma and bile levels of corisin, a microbiota-derived proapoptotic peptide, in patients with severe acute cholangitis. Gut Pathogens, 15(1), Article 59. https://doi.org/10.1186/s13099-023-00587-4
Shi, Q., Abdel-Hamid, A. M., Sun, Z., Cheng, Y., Tu, T., Cann, I., Yao, B., & Zhu, W. (2023). Carbohydrate-binding modules facilitate the enzymatic hydrolysis of lignocellulosic biomass: Releasing reducing sugars and dissociative lignin available for producing biofuels and chemicals. Biotechnology Advances, 65, Article 108126. https://doi.org/10.1016/j.biotechadv.2023.108126
Abdel-Hamid, A. M., D'Alessandro-Gabazza, C. N., Yasuma, T., Walden, K. K. O., Fields, C. J., Gabazza, E. C., & Cann, I. (2022). Complete Genome Sequences of Three Staphylococcus haemolyticus Strains Isolated from the Lung of a TGFb1 Transgenic Mouse with Lung Fibrosis. Microbiology Resource Announcements, 11(4). https://doi.org/10.1128/mra.01176-21