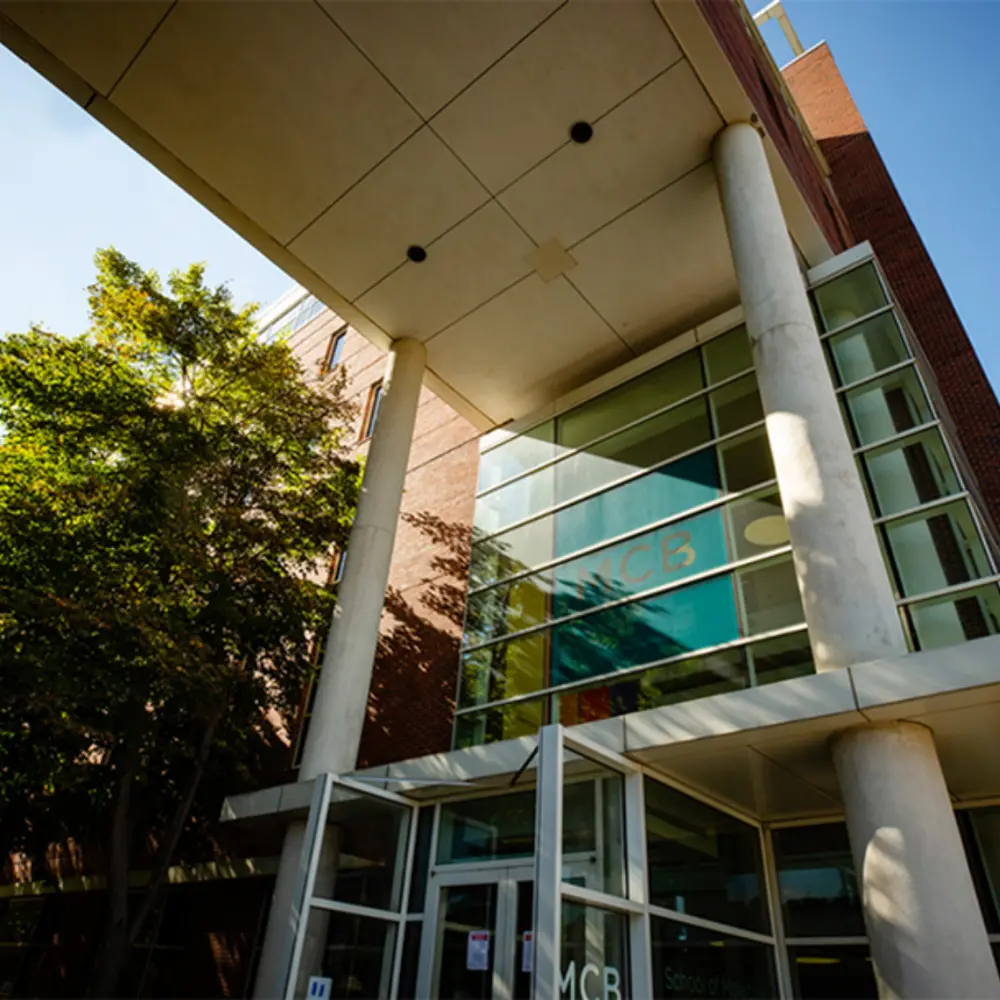
In a new publication, University of Illinois PhD graduate Liguo Zhang of the Belmont laboratory and colleagues introduce an improved version of TSA-seq and use it to demonstrate how changes in gene association with nuclear speckles correlate with changes in gene expression.
The article, “TSA-seq reveals a largely conserved genome organization relative to nuclear speckles with small position changes tightly correlated with gene expression changes,” was published in Genome Research. Zhang, who received his PhD in cell and developmental biology in 2020, and colleagues were joined in this work by collaborators in the laboratory of Jian Ma at Carnegie Mellon University. Cell line comparisons showed that small shifts in relative position towards or away from nuclear speckles strikingly correlate with increased or decreased gene expression, respectively, even comparing human embryonal stem cells with normal fibroblasts or two different cancer cell lines. At the same time, distances to nuclear speckles were remarkably conserved for about 90 percent of the genome.
Nuclear speckles were first discovered over 100 years ago, rediscovered in the 1960s, and then discovered again in the 1990s. Despite being the second largest nuclear body, there remains no clear consensus about their actual function. Two theories have prevailed since the 1990s. The first proposed that nuclear speckles serve primarily as storage sites for factors involved in RNA processing. The second theory proposed that they serve as a hub for active gene expression for a subset of active genes.
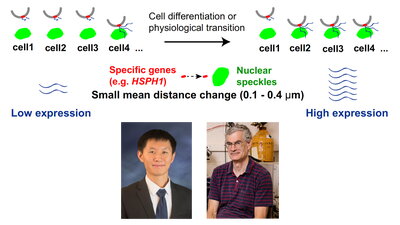
In support of the second model, the Belmont laboratory recently demonstrated amplified expression from Hsp70 heat-shock genes at the HSPA1A/HSPA1B/HSPA1L chromosome locus occurring within several minutes after initial contact with nuclear speckles. Also, the Belmont laboratory developed a new genomic method, TSA-seq, to measure relative distance to nuclear speckles genome-wide. As applied to the erthryoleukemia K562 cell line, TSA-seq revealed a near 100 percent association of certain chromosome regions with nuclear speckles; these Speckle Associated Domains (SPADs) were highly enriched in the most highly expressed genes.
An obvious next question was how chromosomal distances to nuclear speckles varied in different cell types and how these differences correlated with differential gene expression. However, a major limitation of the original TSA-seq method was its requirement for several hundred million cells per measurement. TSA-seq uses the Tyramide Signal Amplification staining method to generate biotin-tyramide free radicals, generated by peroxidases coupled to antibodies. The exponential decay in concentration of these free radicals, spreading radially from the antibody staining target, establishes a “cytological ruler,” allowing estimation of distance of chromosome loci from the staining target by measuring the variation in biotin labeling across the genome. TSA-seq measures the biotin labeling of DNA, rather than chromatin, to avoid a bias by varying protein composition–and labeling–across the genome.
Zhang and colleagues realized that the tyramide labeling efficiency of DNA was much lower than the protein labeling. This led them to deliberately saturate the protein labeling by pushing the peroxidase labeling much further, such that the TSA staining appeared nonspecific in the microscope. However, they realized this allowed them to obtain 10-20-fold higher labeling of the DNA, which remained specific (under-saturated), reducing the number of cells required to 15-30 million per experiment.
Comparison of cell types also suggests that much of the genome may be “hard-wired” in terms of positioning genes near nuclear speckles to facilitate their later gene activation by certain stimuli. For example, previous live-cell imaging of Hsp70 transgenes by the Belmont laboratory had demonstrated that whereas Hsp70 gene induction occurred 2-4 minutes after heat shock when the transgenes already were adjacent to nuclear speckles, induction was slowed by the several or even tens of minutes required for the transgene to move and touch nuclear speckles in cases where the transgenes were located away from nuclear speckles.
Zhang and colleagues discovered that the endogenous Hsp70 locus is among the top 1-2 percent of the genome in speckle proximity, already pre-positioned in multiple cell types very close to nuclear speckles. Moreover, they found this was true for roughly half of all other heat-shock induced genes, while the remaining heat-shock gene loci were positioned with intermediate distance to nuclear speckles prior to heat shock and moved closer after heat-shock. Zhang and colleagues now suggest that this prepositioning of many other genes close to nuclear speckles might facilitate their activation under varying cellular conditions.
Future work will be aimed at better understanding the “logic” of this genomic “hard-wiring” relative to nuclear speckles, using this new TSA-seq 2.0 method to map chromosomal positioning relative to nuclear speckles genome-wide in multiple cell types under varying physiological conditions and developmental stages. Moreover, extension of TSA-seq 2.0 to other nuclear compartments, such as nuclear lamina or nucleoli, will further elucidate nuclear organization and function.