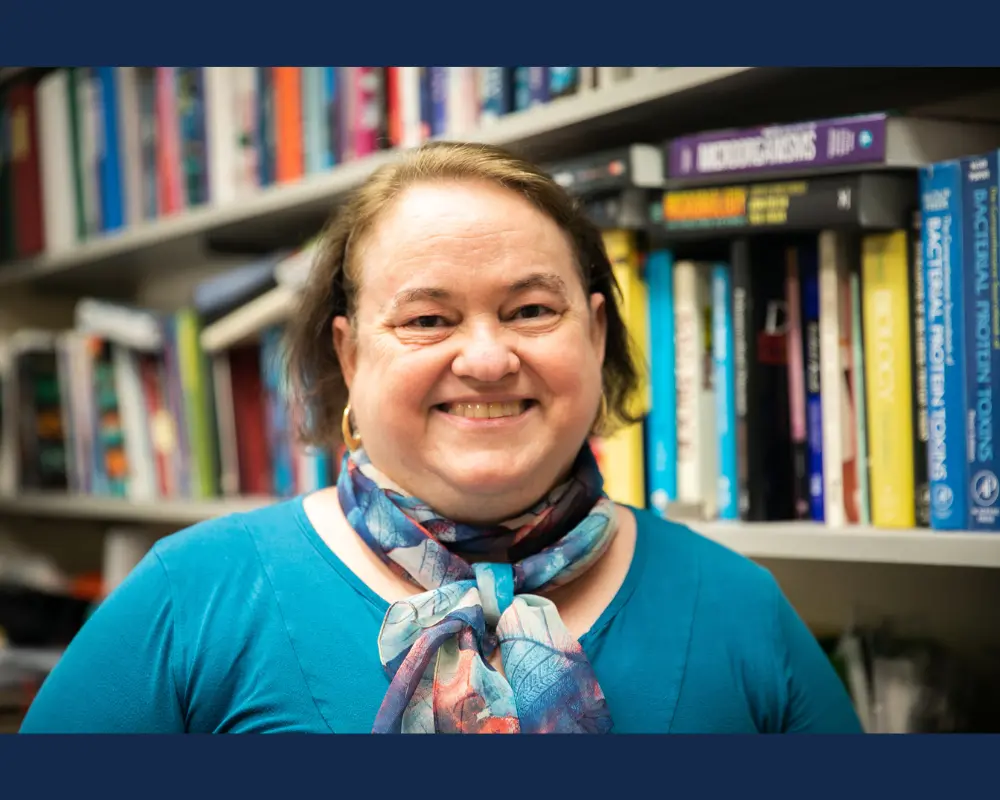
A group of researchers from the University of Illinois has identified a set of rules governing the reaction preferences of a family of G-protein-modifying toxins. G proteins are proteins that regulate cellular processes through interactions between hormone receptors and downstream signaling pathways.
Their findings, published in mBio, will aid scientists in designing bacterial toxin-inspired drug delivery (BTIDD) tools such as anti-cancer therapeutic agents.
“More and more people are using naturally occurring toxins as very specific therapeutics,” said Brenda Wilson, a professor of microbiology and corresponding author of the paper. “The problem is getting the therapeutic cargo delivered into the [cell’s] cytosol efficiently and knowing the rules that are necessary for that to happen. And we’ve recently demonstrated how we can do that for this family of toxins.”
Most bacterial toxins possess little sequence homology, making it difficult for researchers to determine their catalytic mechanisms. One of these toxin types, known as type AB, functions as a cargo-delivery system. Cargo references the toxic material infiltrating a host cell to damage it, while a delivery vehicle consists of the receptor recognition binding domain and the translocation domain, which brings the cargo across the host cell’s membrane and into the cytosol. When a toxin recognizes a host cell receptor, it uses its binding domain to internalize itself into the host cell’s vesicles. Once inside a vesicle, the toxin is trafficked to other cellular compartments.
“That is the significance of these toxins,” Wilson said. “They can deliver their toxic cargo directly into the host cell.”
This ability is a key feature of BTIDD systems, which use naturally occurring toxins to treat everything from cancer to migraines and even scars and wrinkles.
Pharmaceutical companies strive to deliver their biological drugs into a cell’s cytosol, where it can access its target and be most effective. Many companies have been able to bring these drugs to the cells they are targeting, and many can even enter an endosome vesicle. But biologics are vulnerable inside these vesicles, where they are heavily targeted for degradation. Thus, the challenge is escaping vesicles as soon as possible so therapeutic cargo can be delivered directly into the host cell’s cytosol where their targets are located.
“Toxins already know how to do this,” Wilson said. “They’ve been doing it for many moons. And that’s the beauty of using toxins as our platforms for transferring biologic proteins into the cytosol.”
Wilson said that toxins can be used for more than just therapeutic cargo-delivery vehicles; their toxic cargos are potent enzymes that can also be used to manipulate cellular processes. One such example of using toxin cargos is their application as immunotoxins for targeting and killing cancer cells. Several of these anti-cancer immunotoxins have already been approved by the US Food and Drug Administration (FDA) for clinical use. Historically, researchers have struggled to determine the cellular reaction outcomes of the different toxin proteins and engineer them to carry out very specific actions leading to beneficial therapeutic results.
“When we’re using toxins as therapeutic medicines, we need to know exactly what the activity domain is going to do,” Wilson said. “We want to make sure that whatever toxin we’re using will only catalyze the reaction that we specifically want on the substrates and targets that we specifically want. And that is done by determining the rules for these reactions, so we can use protein engineering to conduct modifications that will facilitate a very precise outcome.”
In other words, researchers need to ensure that the biologics they create won’t have detrimental side reactions, like causing cancer rather than curing it.
The group’s first breakthrough for a particular family of G-protein-modifying toxins came in identifying a variant of the cytotoxic necrotizing factor (CNF) that behaved differently than other CNFs. Most CNFs exhibit high homology and were all previously believed to be deamidases, which catalyze the conversion of a glutamine residue to a glutamic acid residue in their G-protein target. But in comparing active sites and cellular activities among these CNFs, the researchers discovered that a variant called CNFx had a preference for transglutamination, a process that attaches a polyamine to a glutamine residue. The group harnessed CNFx as a tool for discovering the hierarchical rules governing reaction preferences in these G-protein-modifying toxins.
“The toxin modules swap all the time, where the cargo is the same while the delivery vehicle is completely different, and vice versa,” Wilson said. “By doing sequence comparisons and directed mutagenesis among these toxins, we were able to establish a series of rules to identify the primary factor in deciding a reaction outcome. It’s like a flowchart that will tell you under which conditions a reaction is likely to be a deamidation or a transglutamination.”
These rules will now allow biologists and pharmaceutical companies to design toxins that are highly specific to their intended target, allowing them to carry out the precise reaction desired.
“These biologics are very powerful,” Wilson said. “But they’re only as powerful as the precision behind them. We can now compare oranges with oranges, instead of oranges with apples. And we now have a set of rules defining why one orange was behaving like an apple.”